|
Citations Index
>>> |
Articles With Citations
to Z. Abou-Assaleh
|
Theoretical Plasma
Physics |
Controlled Thermonuclear Fusion Energy |
|
1995 |
|
|
|
1995 No 03 |
|
Rep. Prog. Phys. 58 (1995) 1-59. Primed in the UK
0034-4885/95/010001+59S59.50 ©
1995 IOP Publishing Ltd
W Arter 1995
Rep. Prog. Phys. 58
1-59
|
"Numerical simulation
of magnetic fusion plasmas"
W Arter
Culham Lab., AEA Technol., Abingdon, UK
Print publication: Issue 1 (January 1995)
". . .
Lastly, it is
worth noting that Fokker-Planck codes have been written to
model inertial confinement fusion experiments, e.g. Andrade et
al (1981), and at least one such (FPl) has then been used to
model
tokamak edge
plasmas (Abou-Assaleh et al 1990). The computational
details are not directly relevant to this review, but the
physical results from FPl are interesting, since they suggest
that the heat transport is not well modelled by corresponding
fluid codes. A comparison with results obtained using particle
methods (section 3.6) would be of interest.
. . ."
|
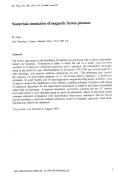 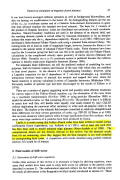 |
|
Abstract
The review
specializes to the modelling of plasmas in a particular type
of fusion experiment, namely the tokamak. Simulation is taken
to imply the use of a model which involves variation in at
least two coordinate directions and is nonlinear, the
nonlinearity invariably being of the advective type.
Developments in the period 1976-1992 are covered under five
main headings, with particle methods constituting the first.
The remaining four concern the solution via mesh-based methods
of (1) the Fokker-Planck equation, (2) drift-wave problems,
(3) edge models and (4) time-dependent magnetohydrodynamic
problems. Care is taken to outline the capabilities of the
currently available software. Progress in the. Design of
numerical algorithms for the mesh-based simulations is found
to have been incremental rather than revolutionary. In
particle simulation, gyrokinetic schemes and the ' delta f'
method have been found to give dramatic gains in some
circumstances. Many of the newer results obtained withstand
comparison with experimental observation, although it has not
always proved possible to reach the extreme conditions found
in tokamaks, especially when three-dimensional effects are
important
|
|
doi:10.1088/0034-4885/58/1/001
URL: http://stacks.iop.org/0034-4885/58/1
|
http://www.iop.org/EJ/abstract/0034-4885/58/1/001/
|
Numerical simulation of magnetic fusion plasma
|
Institute of Physics Online Archive
|
|
|
1995 No 02 |
|
Physics of Plasmas -- August 1995 -- Volume 2, Issue 8, pp.
3100-3105
|
"Self-similar electron distribution,
inverse bremsstrahlung, and heat flux inhibition in high-Z
nonuniform plasmas"
-
S. A. Uryupin,
S. Kato, and
K. Mima
-
Institute of Laser Engineering, Osaka University, Suita, Osaka
565, Japan
(Received 23 June 1994;
accepted 12 April 1995)
Abstract
The
self-similar distribution of electrons is found for a nonuniform
underdense plasma that is heated by an intensive
laser field. The distribution function is flat-topped
for the low-energy electrons. And in
the high-energy region, it has a well-pronounced
high-energy tail. It is also found how the electron
heat flux and the absorption coefficient depend upon
both the ratios of electron mean-free path to the
inhomogeneity scale of effective temperature and of
the oscillation velocity to the thermal velocity. The actual
shapes of electron energy distribution, the heat flux
limitation, and the electromagnetic radiation
absorption rate are given, both for a currentless
plasma and for a plasma with a finite electric current.
©1995 American Institute of Physics.
|
Self-similar electron
distribution, inverse bremsstrahlung, and heat flux inhibition
in high-Z nonuniform plasmas
|
Self-similar electron
distribution, inverse bremsstrahlung, and heat flux inhibition
in high-Z nonuniform plasmas
|
|
1995 No 01 |
|
Physics of Plasmas -- May 1995 -- Volume 2, Issue 5, pp.
1412-1420
|
"Modeling and effects of nonlocal
electron heat flow in planar shock waves"
-
F. Vidal
and
J. P. Matte
-
Institut National de la Recherche Scientifique—Energie et
Matériaux, 1650 Montée Ste. Julie, CP 1020, Varennes, Québec
J3X 1S2, Canada
-
M. Casanova
and
O. Larroche
-
Commissariat à l'Energie Atomique, Centre d'Etudes de
Limeil-Valenton, 94195 Villeneuve St. Georges Cedex, France
(Received 7 November
1994; accepted 4 January 1995)
Abstract
Electron
heat flow was computed in the context of a steadily
propagating shock wave. Two problems were studied: a Mach 8
shock in hydrogen, simulated with an ion kinetic code, and
a Mach 5 shock in lithium, simulated with an Eulerian
hydrodynamic code. The electron heat flow was calculated with
Spitzer–Härm
classical conductivity, with and without a flux limit, and
several nonlocal electron heat flow formulas
published in the literature. To evaluate these, the
shock's density, velocity, and ion temperature profiles
were fixed, and the electron temperature and heat flow
were compared to those computed by an electron
kinetic code. There were quantitative differences
between the electron temperature profiles calculated with
the various formulas. For the Mach 8 shock in hydrogen,
the best agreement with the kinetic simulation was
obtained with the Epperlein–Short delocalization
formula [Phys. Fluids B 4, 2211 and 4190 (1992)], and
the Luciani–Mora–Bendib formula [Phys. Rev. Lett. 55,
2421 (1985)] gave good agreement. For the Mach 5 shock
in lithium, both of these gave good agreement. The earlier
Luciani–Mora–Virmont formula [Phys. Rev. Lett. 51, 1664 (1983)]
gave fair
agreement, while that of San Martin et al. [Phys. Fluids
B 4, 3579 (1992); 5, 1485 (1993)] was even further
off than the classical Spitzer–Härm [Phys. Rev. 89, 977 (1953)]
formula for thermal conduction. To assess the effect of nonlocal
electron heat flow on the shock's hydrodynamics and ion
kinetics, each of the two problems was done with two
different electron heat flow models: the classical
Spitzer–Härm local heat conductivity, and the
Epperlein–Short nonlocal electron heat-flow formula. In spite of
the somewhat different electron temperature profiles, the
effect on the shock dynamics was not important. ©1995
American Institute of Physics.
|
Modeling and effects of nonlocal
electron heat flow in planar shock waves
|
Modeling and effects of nonlocal
electron heat flow in planar shock waves
|
|
|
|
|
Citations Index
>>> |
|